The project relies on three main pillars: data collection, laboratory and field experiment analyses, and chemical and physical modeling processes, detailed below.
FIST PILLAR: HYDROLOGICAL, HYDROGEOCHEMICAL AND SEDIMENT MONITORING
The Hydro4C project has a strong component related to data acquisition and will collect information for at least one hydrological year. Consequently, many of the activities of the first pillar are aimed at collecting and organizing data. For this reason, the project includes a specific activity devoted to design and to implement a scientific data repository to collect and organize the data acquired during the project and those acquired by third parties.
The catchment monitoring network does not include continuous monitoring of the suspended sediment concentration (SSC) which is needed by the project. For this reason, in the Hydro4C project, we will install a multiparametric probe (including the conductivity and the turbidity sensors) in each studied catchment. Turbidimeters must be calibrated through samples collected using depth-integrating samplers such as US DH-59 and/or automatic pumping-type samplers (see following figures) which are already available to the RUs.
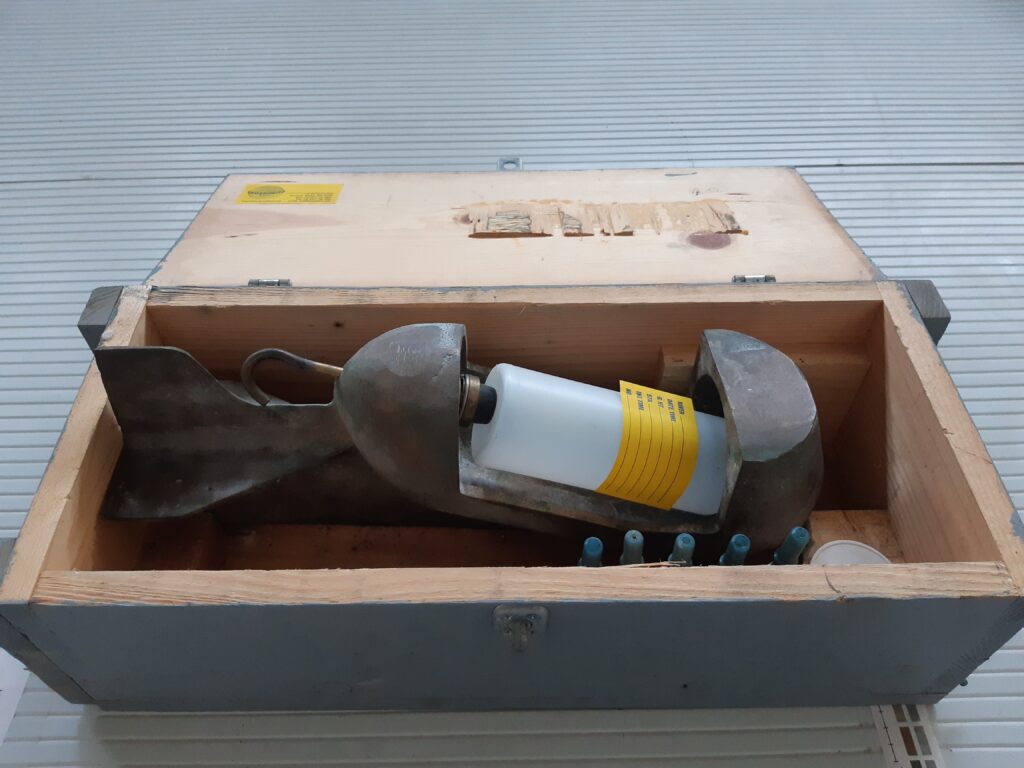
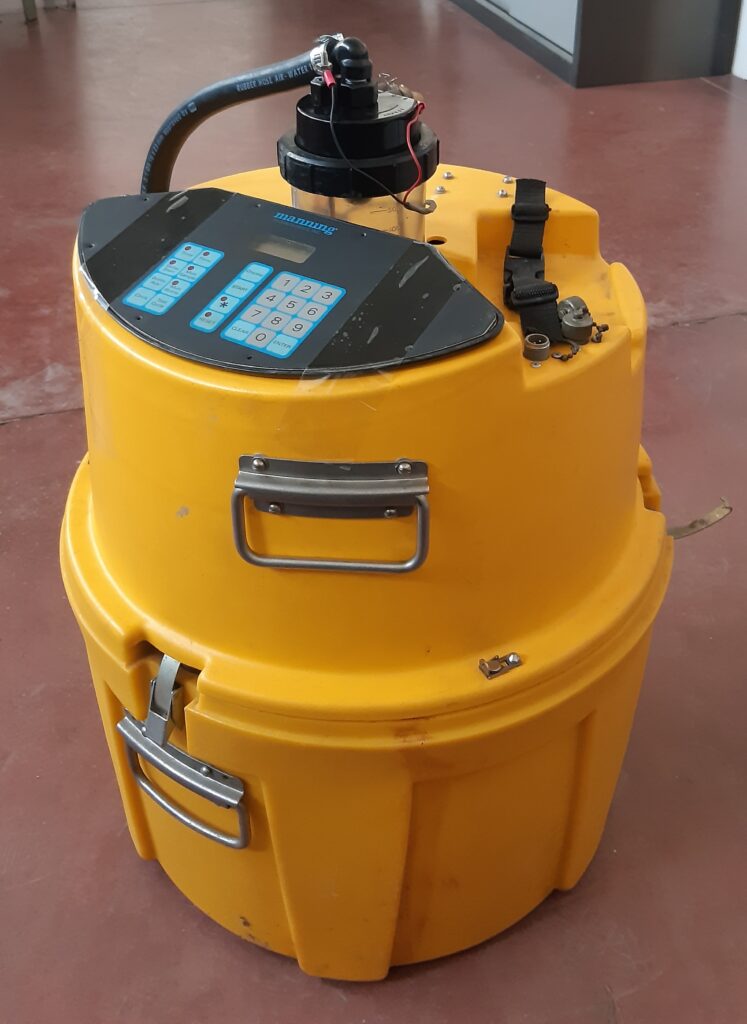
Real-time data of rain and of water levels provided by the network of monitoring sensors and by third-party providers will allow the design and implementation of an automatic system able to activate the researchers and technicians of the RUs who are in charge of collecting waters, dissolved sediment concentration (DSC) and SSC during and immediately after intense meteorological events.
For each sample, temperature, EC, pH, and HCO3 concentration (in the following also defined alkalinity) will be measured in the field. Alkalinity will be determined through portable dosimeters (see following figure) that perform acid titration with HCl 0.1 N using methyl orange as indicator.
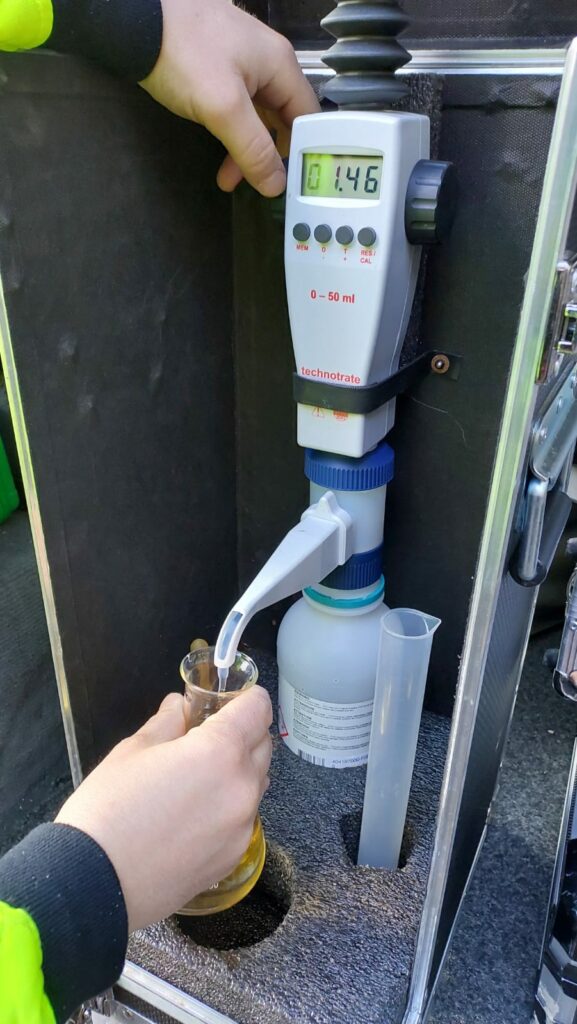
Finally, to derive the abundance of minerals reacting with carbonic acid, rock/soil sampling activities will be implemented. The pilot catchments will be preventively characterized based on both lithology and land cover/use to identify the locations of samples.
SECOND PILLAR: LABORATORY AND METEOROLOGICAL CHARACTERIZATION OF THE THREE PILOT RIVER CATCHMENTS
Aims of the second pillar is (i) the meteorological characterization of the pilot river catchments and (ii) the analyses of the sampled environmental matrices as river waters, suspended sediments, and soils/rock.
Exploiting the data repository, catchments sensors data will be analyzed at different time scales, from runoff events to monthly scale, to derive a meteorological characterization of the pilot river catchments.
Laboratory analyses, the second element of the pillar, will be performed in the sampled environmental matrices, (i.e. river waters, suspended sediments, and soils/rock).
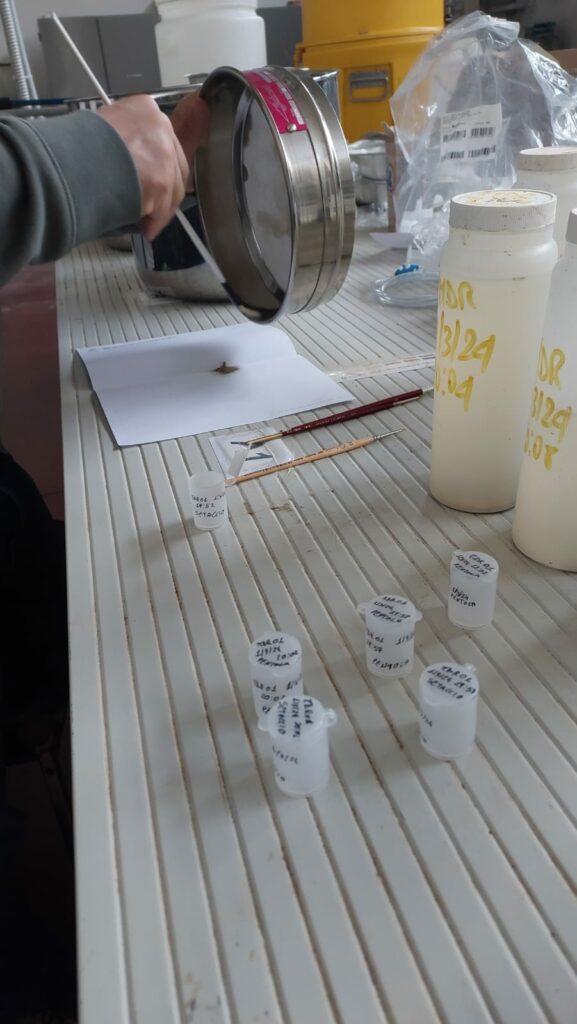
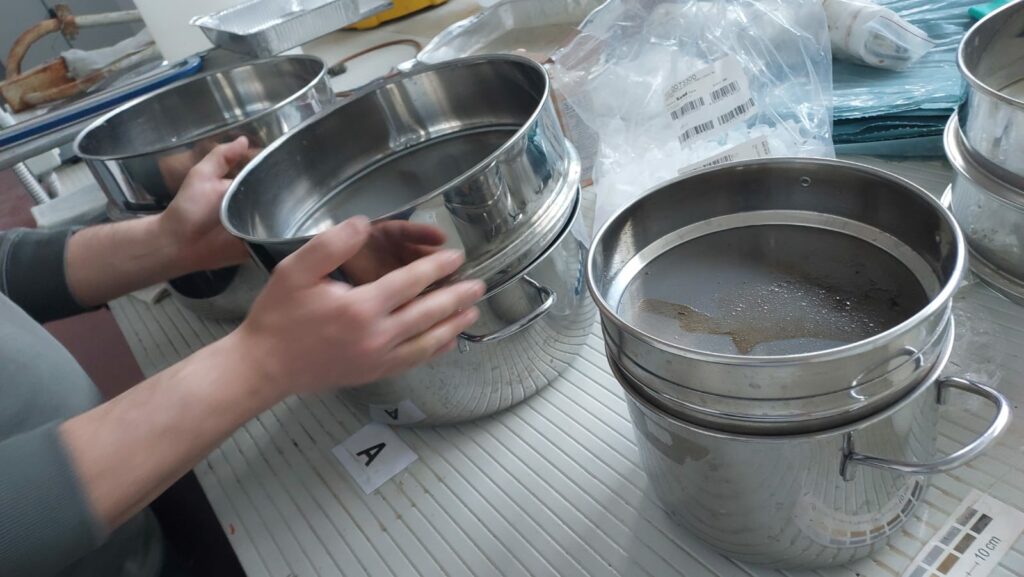
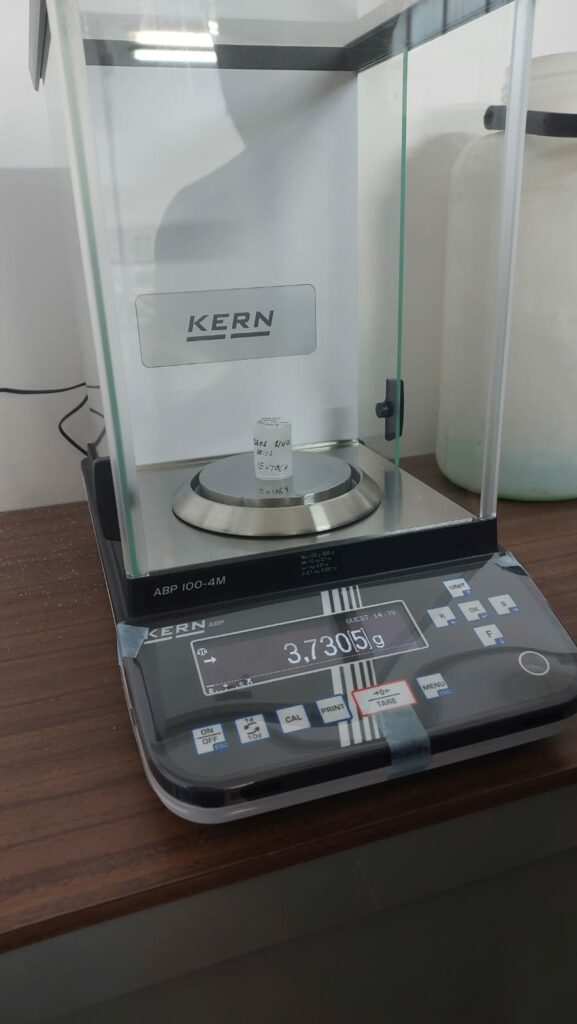
Laboratory activities
THIRD PILLAR: MODELING ACTIVITIES
The third pillar’s goal is to understand the relationships between hydrological catchment processes and atmospheric CO2 consumption. Three different modeling activities will be carried out.
The first one is devoted to the empirical modeling of the relationship between hydrological and geomorphological parameters with the weathering products.
The second one aims to derive empirical relationships between water alkalinity and electrical conductivity.
Finally, combining the results of the previous two activities, Hydro4C will develop models to predict CO2 consumption starting from the simulation of hydrological and geomorphological processes.
Recent studies have highlighted that erosion may be a proxy of atmospheric CO2 consumption ([1,2,3,4,5]). Hydro4C project will investigate the empirical correlation among erosion (evaluated by SSC measurement) and silicate/carbonate weathering products (evaluated by DSC measurement). Moreover, the relationship between EC and SSC will be investigated ([6]).
In the project we will investigate the possible empirical relationship between EC and alkalinity [HCO3] in the three pilot river catchments. A significant correlation between EC and some physicochemical parameters (including alkalinity) was observed by [7]. In the Hydro4C project a relationship between EC and Q will also be searched as already investigated by [8].
The atmospheric CO2 consumption in the three pilot river catchments will be estimated by applying the methodologies well known in the literature (see e.g. [9,10,11]) that make use of HCO3 concentration (i.e. alkalinity) in river waters (the so-called “forward method”).
Specific activities will be performed within the Ussita catchment to determine the CO2 fluxes in a fractured carbonate environment. As highlighted by [12], in a fractured carbonate environment, carbonate weathering is the main source of the hydrogeochemical fingerprint, followed far behind by the weathering of other rocks. In-stream processes such as calcite precipitation can modify the estimation of atmospheric CO2 consumption that could remove over then 70% of HCO3 from stream waters [12]. For this purpose, both hydrogeochemical (i.e. chemical and isotope analyses, see e.g. [13,12,14,15]) and hydrological approaches (e.g. estimation of the river base flow, e.g., [16,17]) will be applied for modeling the atmospheric CO2 consumption in a fractured carbonate environment.
Bibliography
- Erlanger et al 2021, doi:10.1029/2021JF006064.
- Hilton et al 2020, doi:10.1038/s43017-020-0058-6.
- West et al 2005, doi:10.1016/j.epsl.2005.03.020.
- Dixon et al 2012, doi:10.1016/j.crte.2012.10.012.
- Norton et al 2010, doi:10.1016/j.gca.2010.06.019.
- Dai et al 2010, url:https://shorturl.at/gEGOW.
- Shrestha et al 2018, doi:10.1155/2018/8369613.
- Cano-Paoli et al 2019, doi:10.1016/j.scitotenv.2019.05.166.
- Donnini et al 2020, doi:10.1130/B35236.1.
- Hartmann 2009, doi:10.1016/j.chemgeo.2009.03.024.
- Hartmann et al 2009, doi:10.1016/j.gloplacha.2009.07.007.
- Ulloa-Cedamanos et al 2021, doi:10.1016/j.chemgeo.2021.120567.
- Zhong et al 2017, doi:10.1038/srep42944.
- Liu et al 2018, doi:10.1016/j.earscirev.2018.05.007.
- Wang et al 2021, doi:10.1016/j.jhydrol.2020.125710.
- Furey et al 2001, doi:10.1029/2001WR000243.
- Lim et al 2005, doi:10.1111/j.1752-1688.2005.tb03808.x.