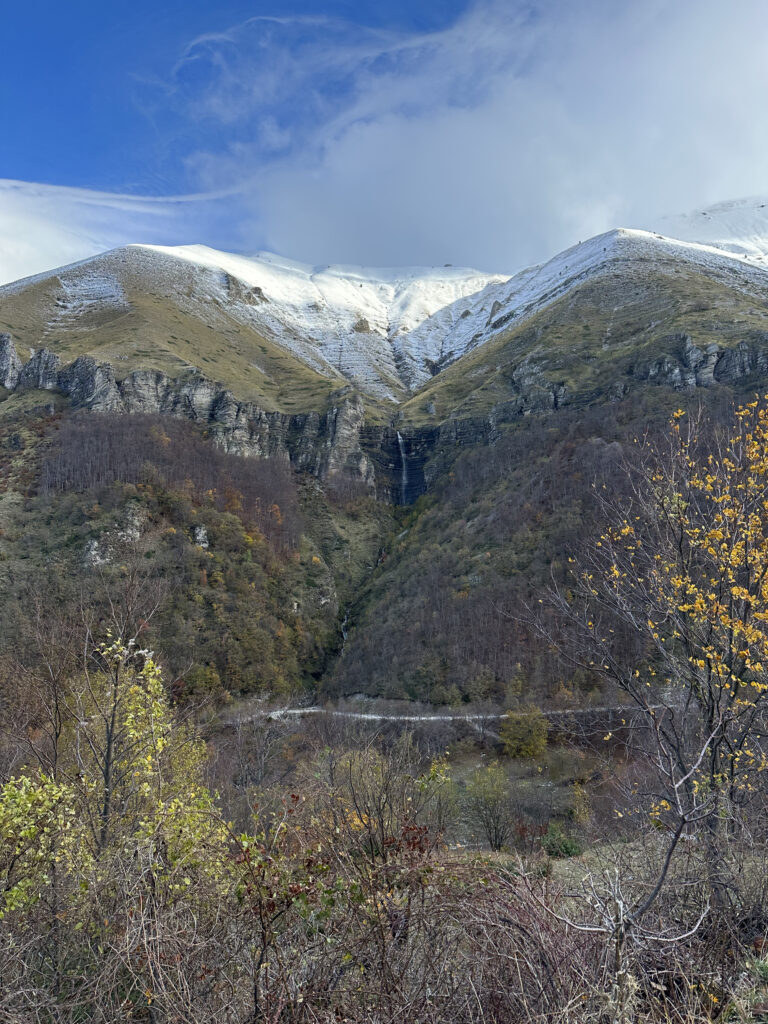
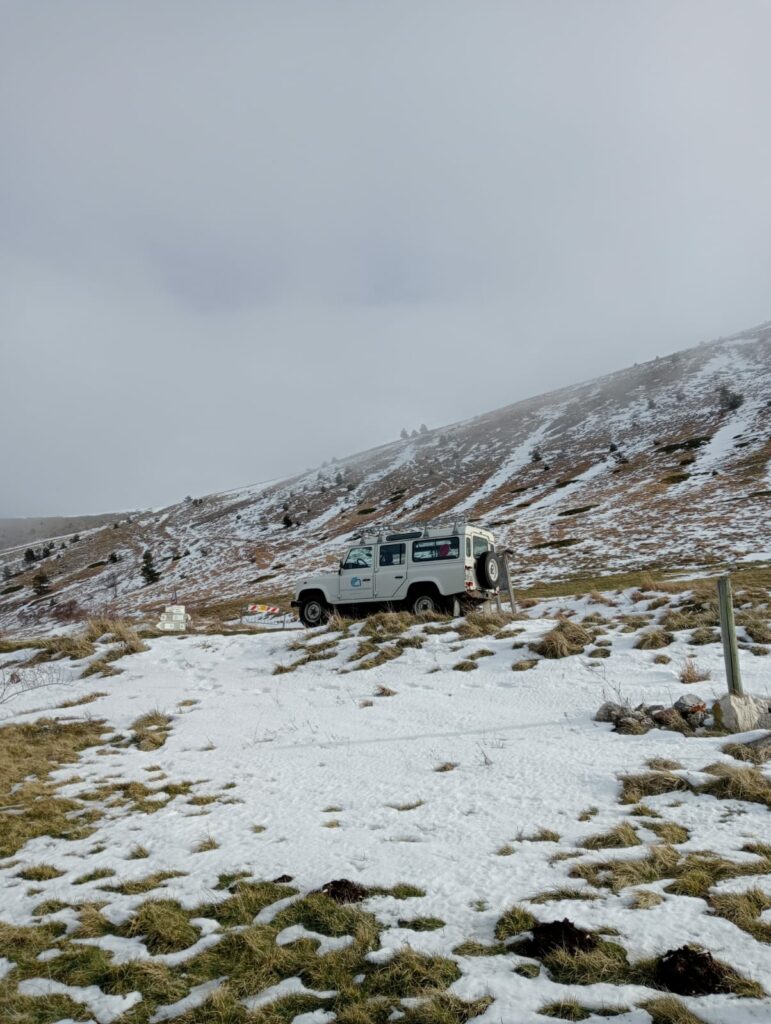
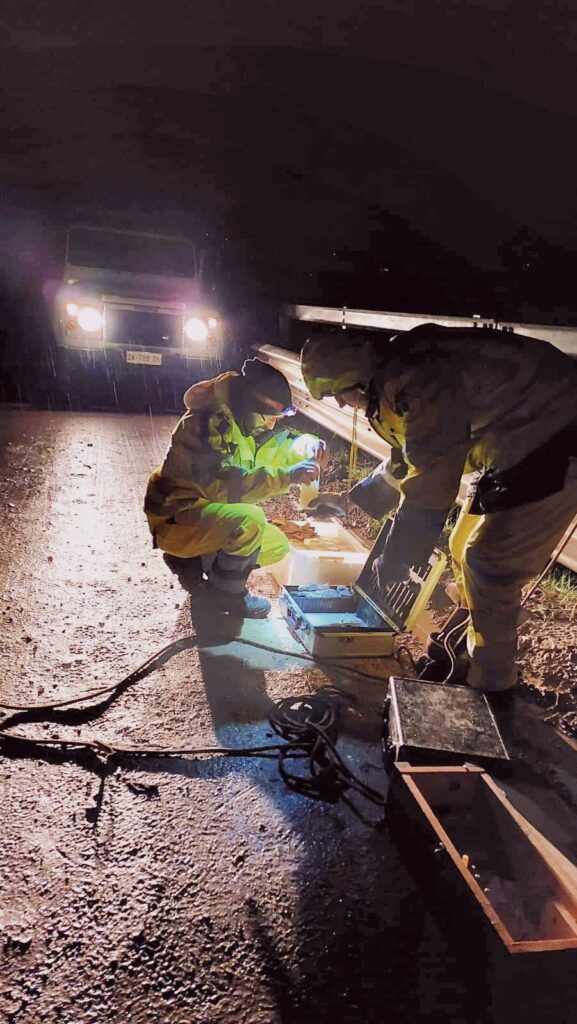
Hydro4C (Hydrological Controls on Carbonate mediated CO₂ Consumption) is a PRIN project (Progetti di Rilevante Interesse Nazionale, PRIN2022 – CUP B53D23007410006, European Union – Next Generation EU) that involves the following research units:
1) CNR-IRPI (Consiglio Nazionale delle Ricerche – Istituto di Ricerca per la Protezione Idrogeologica, Perugia),
2) Università degli Studi di Perugia – Dipartimento di Fisica e Geologia, and
3) Università degli Studi di Firenze – Dipartimento di Scienze e Tecnologie Agrarie, Alimentari, Ambientali e Forestali.
In the following a brief description of the project.
Carbon migrates continuously among oceans, the atmosphere, ecosystems, and the geosphere ([1,2]). Chemical weathering of carbonate and silicate minerals, that consumes atmospheric CO₂ enriching the dissolved load, is an important sink of atmospheric CO₂. Considering the time scale of the phenomena (under or over 1 m.y.), the short-term carbon cycle is distinguished from the long-term carbon cycle (see e.g. [3,4]). In the short-term, carbon is exchanged within surficial systems (i.e. oceans, biosphere, soil, and atmosphere) and the anthropogenic CO₂ production is also taken into account, while in the long-term carbon is exchanged between the geosphere and the ocean-atmosphere system. Since the solutes produced by chemical weathering enrich the river dissolved load, the composition of river waters can be considered as a good indicator of chemical weathering processes ([5,6,7,8,9]). Assuming that the only reactions that occur in the river basins are the dissolution of silicates and carbonates by chemical weathering, knowing the dissolved load, as well as runoff and lithology, it is possible to calculate the atmospheric CO₂consumed by chemical weathering (see e.g. [3,4,6,7,8,9,10,11]). This approach considers that first-order factors that control chemical weathering are lithology and runoff, while second-order factors are (i) hydrological flow path and seasonality, (ii) temperature, (iii) mechanical erosion, and (iv) land cover (see e.g. [7] and reference therein). Within the first-order factors, as highlighted, for instance, by [3,4,10,11], geological maps often give scarce information regarding the chemical and physical nature of the rocks. This lack of information is problematic especially for sedimentary and for metamorphic rocks, leading to uncertainties in the estimates of atmospheric CO₂ consumed by chemical weathering [3]. Moreover, the lithologic maps used to estimate the atmospheric CO₂ consumed by chemical weathering do not consider the land use and the correlated soil processes ([3,4,6,7,8,9,10,11,12]). Within the second-order factors, the relationship between dissolved load and river flow rate was investigated by [13,14] revealing that the variation in ionic concentration is typically much less than the variation in flow rate, calling this behavior chemostatic. [13] highlights that quantifying these correlations in different river basins may help to better understand the scenarios of atmospheric CO₂ concentration in the perspective of climate change. The correlation between erosion and chemical weathering was investigated by [15,16,17,18,19], highlighting how mountain building, enriching physical and chemical weathering, could play a key role in the global carbon cycle. As highlighted by [20], about 40% of global chemical denudation occurs in the steepest 10% area of the world. Consequently, mountainous regions appear to influence the atmospheric CO₂ consumption processes greatly. Moreover, [21] highlight the importance of distinguishing carbonate vs silicate weathering in evaluating CO₂ drawdown, since high erosion rates seem to increase the proportion of solutes from carbonate sources. Recent studies suggest that the role of carbonate weathering in the global carbon cycle cannot be neglected on short timescales, because the fast kinetics of carbonate dissolution makes this process very sensitive to environmental perturbations ([22]). Approximately the weathering rate of carbonate minerals is 10–20 times faster than that of silicates ([5,23]), resulting in a greater CO₂ removal potential in the short timescale, which makes carbonate weathering have a more significant role than silicate weathering in controlling the global carbon cycle in the Anthropocene ([22,24]). In this sense, it is interesting to investigate the role in the global carbon cycle of mixed-carbonate or non-purely silicate lithologies where the carbonate component is non-dominant but non-negligible (see e.g. [3,4,5,6,7,8,9]). Many authors agree in indicating that small percentages of carbonate minerals within mixed lithotypes govern almost all chemical weathering reactions, approximating the behavior of completely carbonate lithologies, at least in the short-term ([3,4,6,7]).
Hydro4C project aims at better understanding and quantifying the hydrological mechanisms that regulate the exchange of CO₂ between the atmosphere and rocks in Mediterranean basins. In particular, the project addresses two fundamental questions:
1) In the short term, is there a significant difference between the CO2 consumption in catchments with mixed sedimentary lithologies versus purely carbonate lithologies?
2) How much atmospheric CO2 consumption is driven by hydrologically governed processes such as erosion and groundwater flow?
Bibliography
- Holland 1978, url: http://shorturl.at/ktzVZ.
- Berner 2003, doi: 10.1038/nature02131.
- Donnini et al 2020, doi: 10.1130/B35236.1.
- Donnini et al 2016, doi: 10.1016/j.gloplacha.2015.10.017.
- Gaillardet et al 1999, doi: 10.1016/S0009-2541(99)00031-5.
- Mackenzie et al 1966, doi: 10.2475/ajs.264.7.507.
- Meybeck 1987, doi: 10.2475/ajs.287.5.401.
- Tardy 1988, url: http://shorturl.at/htwBK.
- Probst 1992, doi: http://shorturl.at/xAUW5.
- Berner et al 2012, url: http://shorturl.at/gBQXY.
- Hartmann 2009, doi: 10.1016/j.chemgeo.2009.03.024.
- Hartmann et al 2009, doi: 10.1016/j.gloplacha.2009.07.007.
- Hartmann et al 2012, doi: 10.1029/2012GC004370.
- Mortatti et al 2003, doi: 10.1016/S0009-2541(02)00349-2.
- Amiotte Suchet et al 2003, doi: 10.1029/2002GB001891.
- Moosdorf et al 2010, doi: 10.1029/2010GC003259.
- Dürr et al 2005, doi: 10.1029/2005GB002515.
- Gibbs et al 1994, doi: 10.1029/94PA01009.
- Clow et al 2010, doi: 10.1016/j.chemgeo.2009.09.014.
- Godsey et al 2009, doi: 10.1002/hyp.7315.
- Erlanger et al 2021, doi: 10.1029/2021JF006064.
- Hilton et al 2020, doi: 10.1038/s43017-020-0058-6.
- Jacobson et al 2003, doi: 10.1130/G19662.1.
- Millot et al 2003, doi: 10.1016/S0016-7037(02)01207-3.
- Riebe et al 2001, doi: 10.1130/0091-7613(2001)029<0511:STAWCC>2.0.CO;2.
- Riebe et al 2004, doi: 10.1016/j.epsl.2004.05.019.
- West et al 2005, doi: 10.1016/j.epsl.2005.03.020.
- Larsen et al 2014, doi: 10.1130/G35136.1.
- Gaillardet et al 2019, doi: 10.1016/j.chemgeo.2018.05.009.
- Meybeck 1986, doi: 10.3406/sgeol.1986.1719.
- Liu et al 2011, doi: 10.1016/j.apgeochem.2011.03.085.